Disclaimer: machine translated by DeepL which may contain errors.
The Rigakubu News
The Rigakubu News, March 2025.
The Frontiers of Research for Undergraduates >
Illuminating K+ ions using a dual approach in chemistry and biology
Takuya Terai, Associate Professor, Department of Chemistry
Robert E. Campbell, Professor, Department of Chemistry
The bodies of living organisms are composed of a variety of proteins, low-molecular-weight metabolites, and ions.
Measuring their temporal and spatial changes is essential for elucidating biological phenomena at the molecular level.
Fluorescent sensors, which emit fluorescence when they bind to a target substance,
are widely used in biological and medical research as useful tools for this purpose.
By directional evolution of complexes of synthetic organic molecules and proteins, we have developed
a high-performance fluorescent sensor that recognizes potassium ions (K+), one of the major intracellular ions, and glows red.
Since red light penetrates biological tissues more easily than blue or green light,
it is expected to be useful in elucidating the role of K+ deep within the organism.
Cellular observation using a microscope is one of the most basic methods for studying living organisms. When the substance of interest is a protein, its location and movement can be analyzed relatively easily by genetically fusing it with a fluorescent protein. However, this method cannot detect the movement of low-molecular-weight metabolites or ions. This is where fluorescent sensors come into their own. These are functional molecules designed to change the intensity and color of fluorescence when they bind to and react with specific targets, and by introducing such sensors into cells, target substances can be visualized (imaged) with high sensitivity in living cells.
However, although research on the development of fluorescent sensors has been conducted since the 1980s, there are surprisingly few targets for which high-performance and practical sensors have been reported. In order to break through this situation, a new basic framework and development method for sensors are considered necessary. One approach that has recently been attracting attention is "chemigenetic," which uses a complex of synthetic small molecules (=chemical substances) and proteins (=gene products).
In this study, we developed a high-performance sensor for K+, the most abundant cation in cells, by utilizing this chemigenetic method. Specifically, they designed a complex sensor that combines a potassium-binding protein (Kbp) from E. coli and JF635, a red fluorescent molecule whose fluorescence intensity changes depending on the surrounding microenvironment (degree of hydrophobicity, presence of hydrogen bonds, etc.), via a protein called HaloTag that selectively covalently binds to small molecules containing chloroalkane. We designed a complex sensor that combines JF635 with a protein called HaloTag, which selectively covalently binds to small molecules with chloroalkane. By building and testing various prototypes, they found a complex molecule that fluoresces in response to K+ concentrations. They further improved the magnitude and brightness of the fluorescence change by repeating the process of creating a population of proteins with slightly different mutations and selecting the best one among them more than 10 times (i.e., directed evolution). Although this process required much time and effort, the sensor HaloKbp-1a635 was not only red fluorescent, which is suitable for tissue-level observation, but also brighter than existing K+ sensors and has a wider range of fluorescence change. This is a result of the combination of the excellent fluorescent properties of synthetic organic molecules and the directable evolution of proteins, which are the strengths of chemical genetic sensors. Furthermore, X-ray crystallographic analysis and live cell imaging of the sensor were successfully performed (Figure).
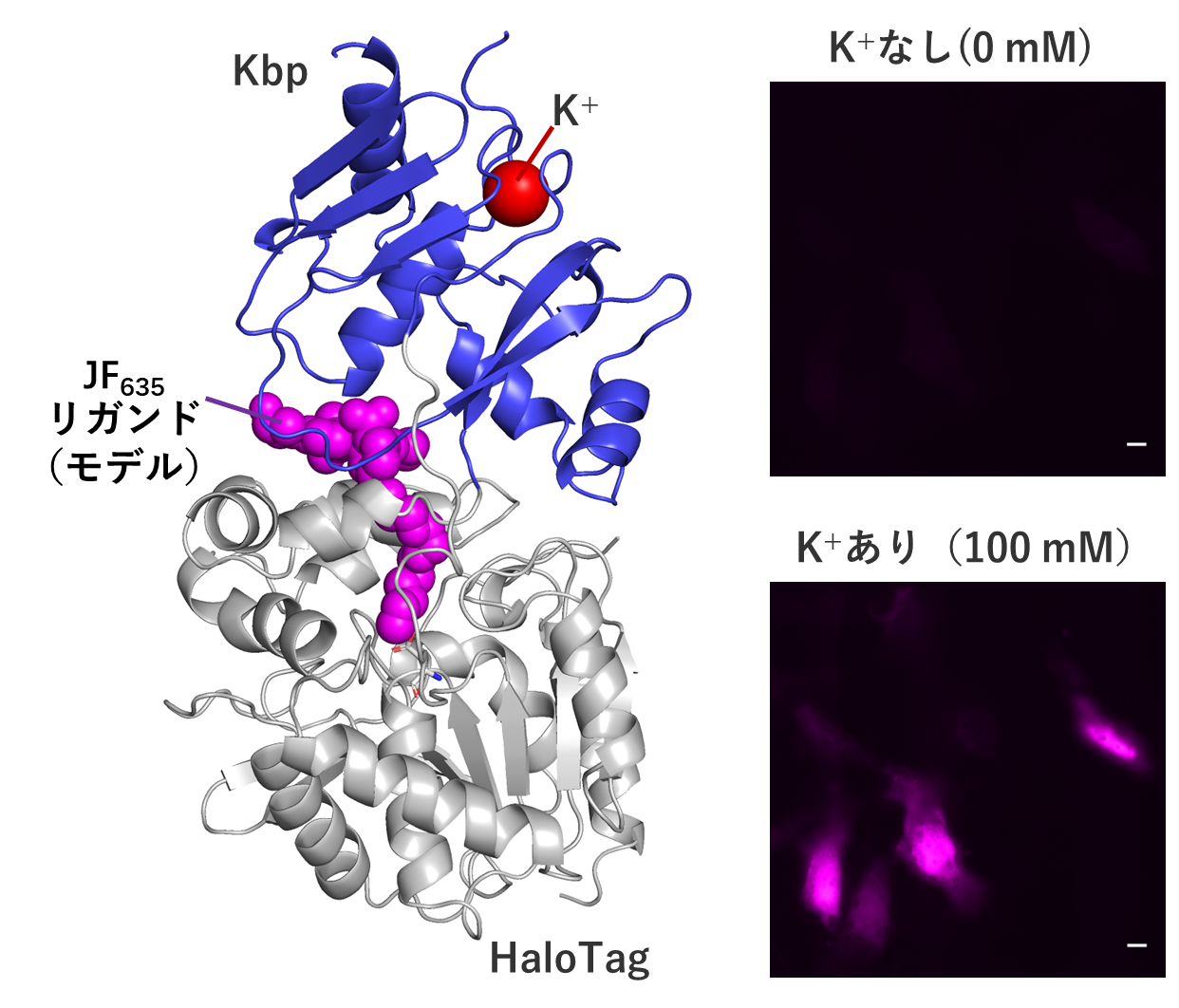
The development of chemical genetics sensors, which can be regarded as a dual approach of chemistry and biology, has just begun worldwide and holds a lot of potential. The findings obtained in this study will be of great use in the development of similar sensors in the future. In addition, K+ is closely related to physiological phenomena such as cell membrane potential regulation and neural activity, as well as to diseases such as hypertension and arrhythmia, and we hope that this sensor will contribute to the development of such research.
The results of this research were published in D. Cheng et al., J. Am. Chem. Soc., 146, 35117(2024). It was also selected for the Journal Supplementary Cover.
(Press release, November 30, 2024)